The Big Bang
Infrared light, along with even longer wavelengths of light called microwaves, has given us direct evidence of the Big Bang as the beginning of time and space.
The Beginning of the Universe
13.7 billion years: that’s the age of the Universe!
When astronomers talk about the "birth and evolution" of the Universe, they are talking about the development of spacetime, with space itself expanding as time moves forward from the creation of the Universe, known as the "Big Bang." Although the "Bang" part of the Big Bang seems to imply that the Universe began with a humongous explosion, an explosion would mean that the Universe -- which started as a singularity, or point of infinite density -- had something to explode into. But what the Big Bang actually means is that the very fabric of space itself came into existence!
At its beginning, the Universe existed in a highly compressed primordial state, filled with an extremely hot, dense form of matter, so hot that not even atoms could exist. As the Universe expanded, however, it also cooled, allowing energy to convert into subatomic particles, including protons, neutrons, and electrons. While protons and neutrons combined to form the first atomic nuclei only a few minutes after the Big Bang, it took thousands of years more for electrons to combine with atomic nuclei and create electrically neutral atoms. The first elements produced were hydrogen and helium, along with traces of lithium and beryllium. Giant clouds made up of those first elements eventually coalesced through gravity to form stars, and those early stars, and subsequent generations of stars, produced most of the heavier elements that we observe in galaxies today.
Evidence for the Big Bang
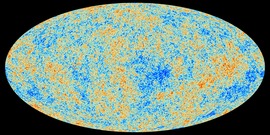
Microwave observations, combined with infrared observations, have given us direct evidence that the Universe evolved from the Big Bang. The first solid evidence was the detection of the Cosmic Microwave Background Radiation (CMBR). Arno Penzias and Robert Wilson received the Nobel Prize in physics in 1978 for discovering the CMBR.
When the Universe was very young and hot, it was made of plasma, which continually emitted and re-absorbed light, preventing the light from traveling through space. Essentially, space was opaque. As it cooled, however, the plasma began to form neutral atoms, which did not absorb light in the same way. The Universe became transparent, and light shone freely.
The CMBR is the light that was emitted by the hot plasma at the moment that the Universe went from opaque to transparent. That light was originally at optical and infrared wavelengths, but thanks to the expansion of the Universe, that light is now primarily in the microwave region of the spectrum.
So how do infrared observations help in detecting the microwave background? Well there are many foregrounds that emit in microwave light hence contaminating our view of the microwave background. So to just see the CMBR it is necessary to remove these foregrounds. Fortuntaely the vast majority of the foreground microwave emittors, mostly dust and gas in our own Galaxy, also emit significantly at infrared wavelengths. So by imaging in both microwave and infrared wavelengths, the foregrounds can be identified and removed, leaving only the microwave background.
Having isolated and studied the CMBR to very high accuracy, it has been shown that the Universe is almost exactly the same temperature in every direction in the sky. For the temperature to be that uniform, scientists have determined that the entire Universe must have once been in the same place - hence the Big Bang.
The CMBR tells us even more. Space telescopes designed to explore the CMBR started with the Cosmic Background Explorer, COBE, which measured the cosmic background radiation of the Universe in infrared and microwave wavelengths across the whole sky. COBE discovered an exact match between the measured background temperature of the Universe and the model (called a blackbody curve) predicted by Big Bang theory. COBE also mapped tiny fluctuations in the temperature of the cosmic background radiation, which track density variations in the early Universe. It is these tiny density variations that are thought to have clumped up to form the Universe's very first galaxies, sparking the progression towards the Milky Way, the formation of the Sun and Earth, and ultimately, us. John Mather and George Smoot won the 2006 Nobel Prize for their work on COBE's precise determination of the temperature and non-uniformity of the CMBR.
COBE was followed by the Wilkinson Microwave Anisotropy Probe (WMAP) and, most recently, the European mission, Planck. Both WMAP and Planck mapped the tiny fluctuations in the background temperature to progressively greater accuracy, showing evidence for a period of very fast growth in the early Universe (called inflation), refining the age of the Universe, and allowing us to determine the composition of the Universe to an astonishing degree of precision.
Composition of the Universe
Planck's detailed map of the oldest light in our Universe has allowed scientists to take the most precise inventory of the Universe's ingredients to date. Normal matter that makes up all the things we think of as the Universe - us, the Earth, the planets, stars and galaxies, contributes just 4.9 percent of the universe's mass and energy. In fact the light generated by normal matter is contained in another background called the Cosmic Infrared Background (CIRB) (see the The Early Universe for more details). The remaining 95.1% of the Universe is made up of dark matter and dark energy.
Dark matter is invisible matter, which doesn't emit or absorb any kind of electromagnetic radiation at any wavelength, and so it is undetectable with our telescopes. Instead, we detect it indirectly by the way that its gravitational pull affects nearby normal matter. It accounts for 26.8 percent of the Universe's mass and energy. The nature of dark matter is still being investigated, and there are several different competing models for the particles that make up dark matter, including weakly interacting massive particles (WIMPs) and axions.
The remaining 68.3% of the Universe is a mysterious force called dark energy, which can't be seen or detected by conventional means, but is thought to be responsible for accelerating the expansion of the Universe. The theory of dark energy came about thanks to a collaboration between the Hubble Space Telescope and many ground based telescopes, which showed that the Universe was expanding more slowly when it was younger than it is today - in other words, the expansion of the Universe is accelerating with time. This was a surprise to astronomers who had expected the expansion to be slowing down as the force of gravity tugged far-flung galaxies back towards each other. The accelerating expansion means that there must be another force, working against gravity, pushing the Universe's expansion ever outwards. This force became known as dark energy, but very little else is known about it. Upcoming missions, NASA's WFIRST and ESA's Euclid, are designed to study dark energy further.
Published: 10 September, 2013